Our Hydrogen Future
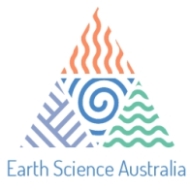
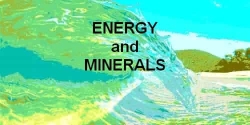
see also
Australia as a Major
Exporter of H2 Hydrogen - questions that need to be addressed
and
Hydrogen Energy in Australia –
Green or Fossil Fuel ?
Australia is being promoted on several levels as a future major
exporter of hydrogen fuel. Not all technologies are equal and CCS
(carbon capture and sequestration) is relied upon to contain some
greenhouse gas emissions from hydrogen production.
The selling point of hydrogen fuel isthat when it is combusted, water
is the only waste product. Hydrogen (H) is also the smallest atom and
the simplest element. Containment during storage and transport is an
issue.
Hydrogen by weight contains three times the energy of petrol, but
because hydrogen is so much lighter (less dense), you need four times
the volume of hydrogen to get the same energy as petrol. However,
hydrogen has some advantages over fossil fuels:
- A fuel-cell car running on hydrogen is 60% to 80% efficient and
achieves between five and 11 times the range (per kilogram of fuel)
than a similar-size petrol-powered car (which is 18-30% efficient).
- Pipeline transport of hydrogen uses three times more volume (and
therefore requires a 20% larger pipe diameter) to supply the same
amount of energy as natural gas. This simple fuel must be extracted
from compounds through processing.
In Australia, some hydrogen fuel is processed from water (H2O)
but most is processed from fossil methane (CH4, commonly
called natural gas). Methane is also found and harvested alongside oil
deposits, coal seams, or fracked from shale formations, or from the
fermentation of organic material.
There are a number of different types of electrolysis devices on the
market, with varying capacities and efficiencies.
To produce 1 kilogram of hydrogen at 100% efficiency:
- add 9 litres of fresh water to a container
- heat to 50 °C to 80 °C.
- insert two electrodes
- place a permeable membrane between the electrodes
- Connect DC electricity from renewable sources
- Oxygen (O2) bubbles off the positive electrode and
- Hydrogen (H2) bubbles off the negative electrode.
The process in reality is 70% to 80% efficient, so a kilogram of
hydrogen will tend to use up to 12 litres of clean water and consume 50
kWh to 55 kWh of renewable electricity.
By comparison, an average Australian house consumes electricity
amounting to 18 kWh per day.
Put simply, the fossil fuel methane (natural gas) is extracted from
underground.
At high temperature, using pure water, it is converted to hydrogen fuel
and carbon dioxide waste.
The carbon dioxide waste is compressed to a liquid/gas state and
injected into geological strata deep in the earth.
The hydrogen fuel is converted to liquid ammonia for export then
converted back to hydrogen fuel or used to make fertiliser at its
overseas destination.
About 7% to 18% of the energy contained in the H2 is lost in
re-conversion.
In the Gorgon Basin of Western Australia, the source natural gas is
mostly methane mixed with about 14% carbon dioxide
--> Using the steam methane
reforming (SMR) process
A first step reacts steam with methane to produce hydrogen and
carbon monoxide and then a second step reacts steam with carbon
monoxide to produce hydrogen and carbon dioxide waste (called the
watershift conversion). Greenhouse emissions come from both input
power and the reaction.
- Steam reformation: CH4 + H2O (+ heat) → CO
+ 3H2
- Water shift conversion: CO + H2O → CO2 +
H2
- So, in total, we have: CH4 + 2H2O → CO2
+ 4H2
- Assuming that there are only these reactions and that they are
complete:
- Four molecules of H2 are created at the same time as
one molecule of CO2
- A mol of H2 weighs 2 g and a mol of CO2
weighs 44 g, so...
- Producing 8 g of hydrogen fuel therefore automatically releases
44 g of waste CO2
- 1 kg of H2 therefore releases 5.5 kg of waste CO2
(at 100% efficiency)
- In practice, in a large hydrogen plant, due to heat losses and
inefficiencies, 1 kg of H2 produces about 9.3 kg of waste CO2
and quantities of waste CH4.
--> Using Pyrolysis of (CH4) Methane
Pyrolysis is the thermal decomposition of materials at elevated
temperatures in an inert atmosphere. Methane pyrolysis decomposition
produces hydrogen with solid elemental carbon particles as a
by-product and no direct carbon emissions.
Under development, it requires high temperatures to break the bonds
of methane to produce hydrogen gas and solid carbon:
CH4 (gas) → C (solid) + 2H2 (gas)
The elemental carbon particles may be used in tyres, added to
concrete, added to agricultural soil or buried in landfill. With
further energy and processing it could possibly be converted to more
valuable graphene.
Graphene is a material composed of pure carbon, similar to graphite
but with characteristics that make it extraordinarily light and
strong. A sheet of one square metre of graphene weighs 0.77
milligrams. Its strength is 200 times greater than that of steel and
its density is similar to that of carbon fibre.
The idea behind CCS is to capture the waste CO2 and inject
it deep into the earth so it can’t pollute the atmosphere or acidify the
oceans.
--> Where to store the CO2
A good geologic storage formation for CCS must meet four main criteria.
- First, for the host formation to receive the CO2, it
must be porous (have spaces) with good permeability (the spaces are
connected)
- Second, the host formation should be at a depth of about two to
three kilometres, with pressures of 200 to 300 bar and temperatures
of 60 °C to 100 °C. This keeps the CO2 in a more compact
liquid/gas state
- Third, it is essential that above the host formation is a
continuous layer of impermeable caprock such as shale. The
liquid/gas CO2 is less dense than the ground water and
will continually try to migrate upwards if it is not blocked
- Finally, the formation must be able to store large volumes of CO2.
--> How is the CO2 kept in place?
Four main mechanisms work together to trap the CO2 in the
formation:
- structural trapping,
- capillary trapping,
- solubility trapping, and
- mineral trapping
--> How long will the CO2 stay underground?
The crust of the earth is dynamic. It erupts, quakes,
moves, bends, breaks, fractures, faults and erodes over time. Eventually
over time the sequestered CO2 will escape. The IPCC
(Intergovernmental Panel on Climate Change) estimates:
- after 100 years, 90% to 99% of sequestered CO2 remains
in place, and
- after 1000 years, sequestered CO2 exceeds 66% to 90%
- the IPCC will not and cannot guarantee that a fluid injected in
the ground will never come out.
Hydrogen fuel production from the electrolysis of water is a battery
technology which uses lots of fresh water and lots of electricity. It is
useful as a fuel “beyond the grid” for bulk transport like ships,
trains, trucks and aircraft where bulky fuel tanks are not a design
issue. It may have a place in adding power to the grid in times of peak
demand, but this begs the question, in a country where fresh water is
scarce, why not use better battery technologies? Hydrogen from natural
gas is a processed fossil fuel. Extraction, conversion and injection and
sequestration processes do not capture all greenhouse gases. How much CO2
and CH4 escapes is currently obscured— it would appear at
least 15% and more realistically 60% is lost before sequestration. After
sequestration, a portion of that which is captured and sequestered will
eventually come back through fugitive emissions.
It’s a gamble whether this kicking the can down the road buys
humankind 100 or 10,000 years
Main Sources
Jin Zhong Zhang, Jinghong Li, Yat Li, Yiping Zhao, 2014, Hydrogen
Generation, Storage, and Utilization, co-published by John Wiley &
Sons, Inc., Hoboken, New Jersey; and ScienceWise Publishing ISBN
978-1-118-14063-5
Garcia L. 2015, Hydrogen production by steam reforming of natural gas
and other nonrenewable feedstocks, in Compendium of hydrogen energy,
Vol. 1 Hydrogen production and purification, éd. Woodhead Publishing,
Kidlington
Herzog, Howard J. 2018, Carbon Capture, The MIT Press Essential
nowledge Series, The MIT Press, Cambridge, MA ISBN 9780262535755
Nuttall William J. , Adetokunboh T. Bakenne, 2020, Fossil Fuel
Hydrogen Technical, Economic and Environmental Potential, Springer
Nature Switzerland AG, ISBN 978-3-030-30907-7 ISBN 978-3-030-30908-4
(eBook)
Havercroft Ian, Macrory Richard & Stewart Richard, 2018, Carbon
capture and storage : emerging legal and regulatory issues, Second
edition, Hart Publishing, Portland, Oregon, ISBN 9781509909605
www.industry.gov.au/sites/default/files/October%202021/
document/australias-long-term-emissions-reduction-plan.pdf Australian
CCS sites map pg 54
climatechangeconnection.org/emissions/co2-equivalents
energy.gov/eere/fuelcells/hydrogen-production-natural-gas- reforming
en.wikipedia.org/wiki/Hydrogen_production
forbes.com/sites/rrapier/2020/06/06/estimating-the-carbon-
footprint-of-hydrogen-production
whatispiping.com/colors-of-hydrogen
large.stanford.edu/courses/2010/ph240/chen1
hydrogene.discoverthegreentech.com/en/production/steam-
methane-reforming
aph.gov.au/About_Parliament/Parliamentary_Departments/
Parliamentary_Library/pubs/rp/rp9899/99rp05#COMP
anz.com/content/dam/anzcom/pdf/institutional/reports/
hydrogen-handbook.pdf
d3n8a8pro7vhmx.cloudfront.net/lockthegate/pages/6525/
attachments/original/1581635517/Fact_sheet_A_tale_of_two_
fuels_-_vs1._pdf.pdf